 |
|
|
back to JAIST/CNSI Workshop main page > |
|
|
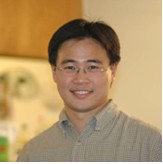 |
|
Eric Chiou, CNSI, UCLA Assistant Professor, Mechanical and Aerospace Engineering |
Plasmonic photothermal single cell surgery |
Approaches that enable the transfer of small objects, such as DNA, RNA, and protein into mammalian cells are numerous and indispensable in biology. Current transfer technologies include endocytosis1, viral infection, lipid encapsulation, fused protein transduction domains, and physical methods such as electroporation5, sonoporation6, optoporation and microinjection. These transfer mechanisms have upper limits, however, on the size of deliverable materials and none can efficiently transfer large objects, such as organelles or bacteria, into somatic cells. To circumvent this size limitation we developed metallic nanostructure guided photothermal microinjection. Our new approach utilizes a metallic thin film coated on the tip of a microcapillary pipette to guide and pattern a laser-induced, high-speed, nanoscale vapor bubble explosion on an adjacent cell plasma membrane. Rapid, reproducible nanobubble expansion and collapse generates a transient, self-resealing, micron-size membrane access port without advancing the pipette into the cell interior. Metallic nanostructure guided photothermal injection delivered biologic and inanimate cargo into multiple mammalian cell types over 3-orders of magnitude in size including DNA, RNA, 30-200 nm polystyrene beads, and micron-sized bacteria with high cell viability (>90%).
References
(1) | Wu, T.H., Kalim, S., Callahan, C., Teitell, M., Chiou, P.Y., Light Image Patterned Molecular Delivery into Live Cells Using Gold Particle Coated Substrates , Optics Express, 2009, 195-6. |
|
|
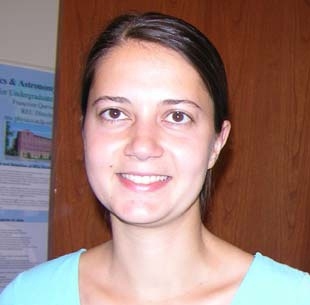 |
|
Dolores Bozovic, CNSI, UCLA Assistant Professor, Physics and Astronomy |
Nano-scale mechanical sensitivity in auditory and vestibular detection |
The inner present a remarkable biological detector that exhibits sensitivity at nanometer levels and better. This process is crucially dependent upon the proper functioning of hair cells, which transduce incoming mechanical displacement into electrical signals. These cells are operant in a viscous medium, but can nevertheless sustain oscillations, amplify incoming signals, and even exhibit spontaneous motility, indicating the presence of an underlying active process that pumps energy into the system. A number of theoretical studies have modeled the hair cells as a nonlinear system with an internal feedback mechanism that can drive it across the Hopf bifurcation and into an unstable regime. We will present latest results on how hair cells self-tune to optimize their sensitivity in response to external mechanical loading and stimulation.
Secondly, we have been developing techniques to simultaneously track movements of multiple hair bundles. With extracellular coupling elements left intact, the hair cells exhibit a significant degree of phase-locking, over the relevant physiological range of stimulus. We believe that inter-cell coupling plays a highly important role in shaping the overall response of the system, enhancing the sensitivity and tuning of the whole sensory epithelium. To explore this effect further, we interface live functional cells with artificial coupling elements and observe the resultant phase-locking.
References
(1) | Ramunno-Johnson, D., Strimbu, C. E., Fredrickson, L., Arisaka, K, and Bozovic, D. Biophys. J. 2009,
96, 1159 |
(2) | Strimbu, C. E., Ramunno-Johnson, D., Fredrickson, L., Arisaka, K, and Bozovic, D. Hear. Res. 2009,
256, 58 |
|
|
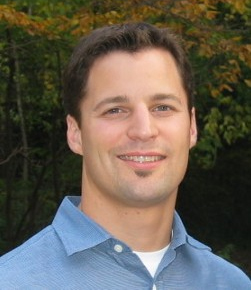 |
|
Eric Hoek, CNSI, UCLA Assistant Professor, Civil and Environmental Engineering |
Nanostructured osmotic membranes for water and energy production |
The basic reverse osmosis (RO) membrane technology that has revolutionized global water treatment strategies is now more than 30 years old. Optimal separation performance, energy efficiency, and fouling resistance of conventional polymeric RO membranes are nearly fully realized, but membrane desalination remains relatively non-selective, energy-intensive, and fouling-prone. These constraints remain in the face of rising worldwide demand for clean water and the sustainability imperatives to control energy use. However, the 'age of nanotechnology' has brought forth entirely new classes of functional materials to be explored. We have already demonstrated nanotechnology-based membrane materials exhibiting major performance advantages; thin film nanocomposite RO membranes are now being commercialized. More recently we are targeting 'smart' membranes with tunable separation properties, antimicrobial reactivity, and self-cleaning surfaces. In this talk, I will discuss a few specific examples of how the union of nanotechnology and membranes is already shaping the future of water treatment.
|
|
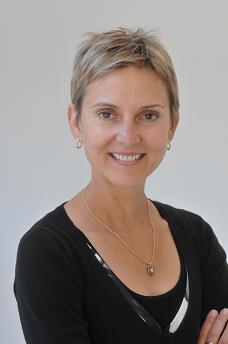 |
|
Diana Huffaker, CNSI, UCLA Associate Professor, Electrical Engineering |
Atomic assembly processes in crystalline III-V nanostructures |
In this presentation we describe our work in III-V nanostructures including quantum dots and nanopillars formed by both directed and random processes. We are particularly interested in controlling material composition and nanoheterostructure formation. We are therefore interested in atomic assembly processes. We report a very flexible approach to nanowire synthesis that enables a wide-range of material combinations along with pre- defined nanowire placement. Specifically, we have demonstrated formation of both GaAs and InGaP nanopillars on several nanopatterned substrates including GaAs, silicon, and SiO2 in the self-catalyzed growth mode. This hybrid approach combines favorable features of both catalyzed and directed assembly (patterned) methods, providing flexibility for material systems, nanopillar placement, and free from hassles of foreign metal particles.
The self-catalyzed growth of both III-As and III-P nanopillars, where Ga droplets, formed in-situ, are used to catalyze subsequent nanopillar growth. We have demonstrated that through the control of growth conditions Ga droplets preferentially form only within pattern openings in the masked SiO2 surface. Spherical Ga catalytical particles are observed atop vertically aligned, highly faceted, and downward tapered GaAs nanopillar bodies grown out of openings of nanopatterned GaAs (111)B and Si (111) substrates. On the other hand, nanopillars grown from Ga droplets atop an SiO2 surface are not aligned and seem to point to random directions, and are not as faceted. Phenomenon similar to Ostwald ripening is observed, where Ga droplets that grow into nanopillars expand in diameter at the expense of neighboring droplets, which become smaller in size or turn into clusters. The TEM and EDS are employed to study the crystalline structure and composition of these nanopillars. While uncatalyzed, patterned nanopillars are characterized by stacking faults along the growth direction that result from the formation of rotational twins, the self-catalyzed pillars are fault-free. Room-temperature and low- temperature photoluminescence of these nanopillars are characterized and studied. Electrical properties including carrier dynamics are analyzed through two schemes: direct probing of attached nanopillars through an Au-coated STM tip, and planar placement of dispersed nanopillars with patterned metal contacts.
|
|
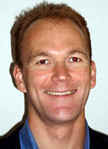 |
|
Vidvuds Ozolins, CNSI, UCLA Associate Professor, Materials Science and Engineering |
Challenges in computational modeling of energy materials |
General adoption of several promising renewable energy technologies depends on the discovery and development of revolutionary new materials. For instance, use of hydrogen as a vehicular fuel depends on the ability to store hydrogen at high volumetric and gravimetric densities, as well as on the ability to extract it at sufficiently rapid rates. Among the various hydrogen storage options (such as compressed gas, chemical liquid hydrides), solid-state storage of hydrogen in materials has attracted particular attention due to the very high storage densities and favorable thermodynamics that can be achieved in these systems. Similarly, waste heat recovery and electricity generation via the solar thermal route require high-temperature bulk thermoelectrics with a high figure of merit (ZT) and high thermal stability, which can be achieved in nanostructured semiconductor alloys. Finally, next-generation nuclear energy technologies depend on the ability to maintain the physical integrity of the nuclear fuel capsules subject to prolonged periods of radiation damage. We will show how first-principles theoretical calculations based on the fundamental physics theories of quantum mechanics and statistical mechanics can be used as a valuable tool for understanding and predicting novel energy materials. Recent studies in our group have used density-functional theory (DFT) calculations to (i) predict crystal structures of new solid-state phases, (ii) determine phase diagrams and phase transformation/reaction pathways, and (iii) study microscopic kinetics of diffusion, energy storage and release.
|
|